Science engineering practices encompass the methodologies and approaches scientists and engineers utilize to investigate, understand, and solve problems. These practices include observing, asking questions, forming hypotheses, experimenting, analyzing data, and developing models.
Science engineering practices are central to scientific and technological advancements, fostering critical thinking, problem-solving abilities, and innovation. Historically, they have contributed to groundbreaking discoveries and technological breakthroughs, shaping our understanding of the world.
In the main article, we will delve deeper into the various science engineering practices, exploring their significance, benefits, and applications in different scientific disciplines and engineering fields.
Science Engineering Practices
Science engineering practices encompass a set of methodologies and approaches that scientists and engineers employ to investigate, understand, and solve problems. These practices form the backbone of scientific and technological advancements, fostering critical thinking, problem-solving abilities, and innovation. Here are eight key aspects that highlight the multifaceted nature of science engineering practices:
- Observation
- Questioning
- Hypothesis Formation
- Experimentation
- Data Analysis
- Model Development
- Communication
- Collaboration
These aspects are interconnected and interdependent. Observation leads to questioning, which in turn drives hypothesis formation. Experimentation allows for data collection and analysis, leading to the development of models that explain and predict phenomena. Communication and collaboration are crucial for sharing knowledge, building upon existing research, and fostering innovation. Together, these practices form a cohesive framework that enables scientists and engineers to make progress in their respective fields.
Observation
Observation is a fundamental aspect of science engineering practices, serving as the foundation for scientific inquiry and technological advancements. It involves the active acquisition of information through the senses, often guided by prior knowledge or research questions.
- Direct Observation: Involves gathering information through observation of phenomena or processes, using tools such as telescopes, microscopes, or sensors.
- Indirect Observation: Involves inferring information about unobservable phenomena or processes by analyzing indirect evidence or data, such as studying fossils to understand ancient life or using remote sensing to monitor environmental changes.
- Qualitative Observation: Focuses on describing and categorizing observed phenomena or processes based on their characteristics, such as color, shape, or behavior.
- Quantitative Observation: Involves measuring and recording numerical data to quantify observed phenomena or processes, providing precise and objective information.
Observation plays a crucial role in science engineering practices by providing the raw data and information upon which all subsequent analyses, interpretations, and technological developments are based. It allows scientists and engineers to identify patterns, formulate hypotheses, and design experiments to investigate the natural world and solve engineering challenges.
Questioning
Questioning is a crucial aspect of science engineering practices, driving the entire process of scientific inquiry and technological innovation. It involves asking probing questions to challenge existing knowledge, identify gaps, and seek deeper understanding of the natural world and engineering problems.
Effective questioning in science engineering practices often begins with observation and curiosity. By carefully observing phenomena or processes, scientists and engineers identify patterns, anomalies, or areas of uncertainty that spark questions. These questions can range from simple and specific to complex and open-ended, guiding the direction of research and development.
The significance of questioning in science engineering practices lies in its ability to uncover new knowledge, challenge assumptions, and drive progress. By asking the right questions, scientists and engineers can identify areas for further investigation, develop innovative solutions, and push the boundaries of human understanding.
Hypothesis Formation
Hypothesis formation is a pivotal aspect of science engineering practices, serving as a bridge between observation and experimentation. It involves proposing an explanation for a phenomenon or problem, based on prior knowledge, observation, and logical reasoning.
A well-formulated hypothesis is testable, meaning it can be supported or refuted through experimentation. It should be specific, clear, and concise, providing a precise prediction that can be empirically verified. The process of hypothesis formation is iterative, involving refinement and modification as new data and evidence emerge.
The significance of hypothesis formation in science engineering practices lies in its role as a guide for experimentation and a means to advance knowledge. By proposing a hypothesis, scientists and engineers can design experiments to test their predictions, collect data, and analyze results to support or reject their initial assumptions. This process leads to the accumulation of scientific knowledge and the development of new technologies.
In the field of medicine, for example, hypothesis formation has played a crucial role in the development of vaccines and treatments for diseases. By proposing hypotheses about the cause and spread of diseases, scientists have been able to design experiments to test their ideas and develop effective interventions.
Hypothesis formation is not limited to scientific research; it is also essential in engineering design and problem-solving. Engineers use hypotheses to propose solutions to technological challenges and design experiments to test their effectiveness. This iterative process of hypothesis formation, experimentation, and refinement is fundamental to the development of new products, materials, and technologies that improve our lives.
In summary, hypothesis formation is a cornerstone of science engineering practices, providing a framework for scientific inquiry and technological innovation. It involves proposing testable explanations for phenomena or problems, guiding experimentation, and advancing knowledge. Its importance extends beyond scientific research, playing a vital role in engineering design and problem-solving, ultimately contributing to the progress of society.
Experimentation
Experimentation lies at the heart of science engineering practices, serving as the primary means to test hypotheses, gather data, and validate or refute scientific and engineering claims. It involves designing and conducting controlled experiments to observe and measure the effects of manipulating variables, while minimizing external influences.
The significance of experimentation in science engineering practices cannot be overstated. It provides a systematic and objective approach to investigating phenomena, testing hypotheses, and advancing knowledge. By carefully controlling experimental conditions and collecting quantitative data, scientists and engineers can gain insights into cause-and-effect relationships, identify patterns, and develop evidence-based conclusions.
Real-life examples abound that illustrate the power of experimentation in science engineering practices. In the field of medicine, clinical trials are essential for evaluating the safety and efficacy of new drugs and treatments. Engineers rely on experimentation to test the strength and durability of materials, the efficiency of energy systems, and the performance of new technologies.
The practical significance of understanding the connection between experimentation and science engineering practices extends far beyond the laboratory. It enables scientists and engineers to develop new products, materials, and technologies that address real-world problems and improve our lives. It also fosters critical thinking, problem-solving skills, and a deep understanding of the scientific method.
In conclusion, experimentation is an indispensable aspect of science engineering practices, providing a rigorous and systematic approach to testing hypotheses, gathering data, and advancing knowledge. Its importance lies in its ability to validate or refute claims, identify cause-and-effect relationships, and contribute to the development of new technologies that benefit society.
Data Analysis
Data analysis is an essential aspect of science engineering practices, providing the means to interpret and draw meaningful conclusions from experimental data. It involves the systematic examination and processing of data to identify patterns, trends, and relationships that would otherwise remain hidden.
- Data Visualization: Converting raw data into visual representations, such as graphs, charts, and maps, to make patterns and trends more easily identifiable.
- Statistical Analysis: Applying statistical techniques to analyze data, including measures of central tendency, dispersion, and hypothesis testing, to draw inferences about the underlying population.
- Machine Learning: Using algorithms to identify patterns and trends in data, enabling computers to learn from data without explicit programming.
- Data Mining: Extracting knowledge and insights from large datasets, often using advanced computational techniques, to uncover hidden patterns and relationships.
Data analysis plays a crucial role in science engineering practices by providing evidence to support or refute hypotheses, validate models, and draw conclusions. It enables scientists and engineers to make informed decisions, develop new theories, and create innovative technologies that benefit society.
Model Development
Model development is a cornerstone of science engineering practices, providing a structured and systematic approach to representing, understanding, and predicting complex phenomena.
- Conceptual Models: Simplified representations of a system or process, focusing on key concepts and relationships. They aid in understanding the underlying mechanisms and making qualitative predictions.
- Mathematical Models: Use mathematical equations to represent and analyze systems. They allow for precise predictions and simulations, enabling engineers to optimize designs and scientists to understand complex interactions.
- Computational Models: Implemented on computers, these models simulate complex systems and processes. They provide insights into dynamic behaviors and allow for extensive experimentation and optimization.
- Physical Models: Tangible representations of systems, such as scale models of buildings or wind tunnels. They enable physical testing and experimentation, providing valuable data for design and analysis.
Model development plays a crucial role in science engineering practices, as it allows scientists and engineers to:
- Gain insights into complex systems and processes
- Make predictions and forecasts
- Optimize designs and solutions
- Communicate scientific and engineering knowledge
Communication
Communication is an integral aspect of science engineering practices, serving as a critical bridge between scientific discovery and technological innovation, and the broader society.
Effective communication enables scientists and engineers to share their research findings, exchange ideas, collaborate on projects, and disseminate knowledge to the public. It takes various forms, including scientific papers, conference presentations, technical reports, and public outreach activities.
Clear and concise communication is essential for ensuring that scientific and engineering knowledge is accurately conveyed, understood, and utilized by diverse audiences, including peers, policymakers, industry professionals, and the general public. Miscommunication can lead to misunderstandings, errors, and a lack of trust in science and technology.
Moreover, effective communication is crucial for fostering collaboration and knowledge-sharing among scientists and engineers. By sharing their findings and ideas, researchers can build upon each other’s work, avoid duplication of effort, and accelerate the pace of scientific and technological progress.
In summary, communication is an essential component of science engineering practices, enabling the dissemination of knowledge, fostering collaboration, and building trust with the public. Scientists and engineers must be skilled communicators, capable of effectively conveying complex technical information to a wide range of audiences.
Collaboration
Collaboration is a fundamental aspect of science engineering practices, fostering a synergistic environment that drives innovation and the advancement of knowledge. It involves scientists and engineers working together, sharing ideas, expertise, and resources to achieve common goals that may be beyond the reach of individuals working alone.
Collaboration plays a pivotal role in scientific discovery and technological development. By combining diverse perspectives, skills, and knowledge, teams of scientists and engineers can tackle complex problems, accelerate the pace of research, and produce more innovative solutions. Collaborative efforts have led to groundbreaking achievements in fields such as space exploration, medical research, and the development of new materials and technologies.
Real-life examples abound that illustrate the power of collaboration in science engineering practices. The Human Genome Project, a global collaboration involving thousands of scientists, revolutionized our understanding of genetics and medicine. Similarly, the development of the World Wide Web, a collaborative effort between scientists at CERN and other institutions, has transformed global communication and information sharing.
Understanding the connection between collaboration and science engineering practices is of great practical significance. It highlights the importance of fostering collaborative environments in research institutions, universities, and industries. By promoting teamwork, open communication, and the sharing of resources, organizations can create conditions that nurture innovation and drive scientific and technological progress.
In conclusion, collaboration is an indispensable component of science engineering practices, enabling the pooling of expertise, perspectives, and resources to achieve ambitious goals and advance the frontiers of knowledge and technology. Its significance extends beyond the laboratory, as collaborative approaches are essential for addressing complex societal challenges and driving economic growth.
Science Engineering Practices FAQs
This section addresses frequently asked questions (FAQs) about science engineering practices, providing concise and informative answers to common concerns or misconceptions.
Question 1: What are science engineering practices?
Science engineering practices encompass a set of methodologies and approaches that scientists and engineers utilize to investigate, understand, and solve problems. These practices include observing, questioning, forming hypotheses, experimenting, analyzing data, and developing models.
Question 2: Why are science engineering practices important?
Science engineering practices are central to scientific and technological advancements, fostering critical thinking, problem-solving abilities, and innovation. Historically, they have contributed to groundbreaking discoveries and technological breakthroughs, shaping our understanding of the world.
Question 3: How are science engineering practices used in real-world applications?
Science engineering practices are applied across a wide range of fields, including medicine, engineering, and environmental science. For example, scientists use observation and experimentation to develop new medical treatments, engineers apply mathematical modeling to design bridges and buildings, and environmental scientists use data analysis to monitor and mitigate pollution.
Question 4: What are the benefits of incorporating science engineering practices into education?
Integrating science engineering practices into education provides numerous benefits for students. It enhances their problem-solving skills, critical thinking abilities, and creativity. Moreover, it fosters a deeper understanding of the scientific process and the role of science and technology in society.
Question 5: How can I learn more about science engineering practices?
There are various resources available to learn more about science engineering practices. These include online courses, workshops, and books. Additionally, many educational institutions offer programs that incorporate science engineering practices into their curricula.
Question 6: What are some examples of science engineering practices?
Examples of science engineering practices include designing and conducting experiments, analyzing data, building models, and communicating scientific findings. These practices are used by scientists and engineers to investigate natural phenomena, solve problems, and develop new technologies.
In summary, science engineering practices are essential for advancing scientific knowledge and technological innovation. By understanding and applying these practices, we can better address complex challenges and shape a better future.
Transition to the next article section:
Science Engineering Practices Tips
To effectively implement science engineering practices in various fields and educational settings, consider the following tips:
Tip 1: Foster a Culture of Inquiry
Encourage active questioning, critical thinking, and exploration to nurture a mindset that values asking questions, seeking evidence, and engaging in the scientific process.
Tip 2: Design Hands-on Learning Experiences
Provide opportunities for students and researchers to engage in hands-on activities, experimentation, and real-world problem-solving to reinforce understanding and develop practical skills.
Tip 3: Integrate Technology for Data Analysis and Modeling
Leverage technology tools and software to assist in data analysis, modeling, and visualization, enabling deeper insights and more efficient problem-solving.
Tip 4: Promote Collaboration and Communication
Facilitate collaboration among students and professionals from diverse disciplines to encourage knowledge-sharing, cross-pollination of ideas, and effective communication of findings.
Tip 5: Emphasize Ethical Considerations
Incorporate discussions on ethical implications, responsible conduct of research, and the potential societal impacts of scientific advancements to foster a sense of responsibility and ethical decision-making.
Tip 6: Seek Professional Development Opportunities
Encourage educators and practitioners to participate in workshops, conferences, and online resources to stay updated on best practices and innovative approaches in science engineering practices.
Tip 7: Align with Educational Standards and Frameworks
Ensure that science engineering practices are aligned with national and state educational standards and frameworks to maintain rigor and ensure coherence across curricula.
By incorporating these tips, individuals and institutions can effectively harness the power of science engineering practices to advance knowledge, solve complex problems, and prepare future generations for success in science, technology, engineering, and mathematics (STEM) fields.
Transition to the article’s conclusion:
Conclusion
Science engineering practices lie at the heart of scientific discovery and technological advancements. Through observation, questioning, experimentation, and analysis, scientists and engineers have unlocked the mysteries of the natural world and developed innovative solutions to complex problems.
This article has explored the multifaceted nature of science engineering practices, highlighting their importance in fostering critical thinking, problem-solving abilities, and creativity. By embracing these practices, we empower individuals to actively engage with the world around them, driving progress and shaping a better future for all.
Youtube Video:
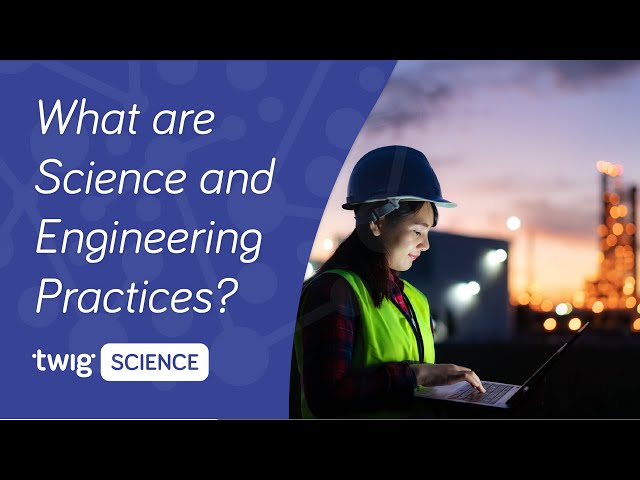