Si Sige Diode Band Diagram
A SiGe diode band diagram is a graphical representation of the energy bands in a SiGe diode. It shows the conduction band, valence band, and the bandgap between them. The band diagram can be used to understand the electrical properties of the diode, such as its forward bias and reverse bias characteristics.
To create a SiGe diode band diagram, the following steps can be followed:
- Draw the conduction band and valence band of the SiGe diode.
- Calculate the bandgap energy of the SiGe diode.
- Draw the Fermi level in the SiGe diode.
- Label the forward bias and reverse bias regions of the SiGe diode.
The SiGe diode band diagram can be used to understand the following:
- The forward bias characteristics of the SiGe diode.
- The reverse bias characteristics of the SiGe diode.
- The breakdown voltage of the SiGe diode.
Tip 1: Use a consistent energy scale for all of the band diagrams.Tip 2: Label all of the important features of the band diagram, such as the conduction band, valence band, bandgap, and Fermi level.Tip 3: Use different colors to represent different materials. For example, you could use blue to represent Si and red to represent Ge.Tip 4: Make sure that the band diagram is clear and easy to understand.Tip 5: Use the band diagram to explain the electrical properties of the diode.
Si Sige Diode Band Diagram
A SiGe diode band diagram is an essential tool for understanding the electrical properties of a SiGe diode. It can be used to visualize the energy bands of the diode, and to understand how these bands are affected by different factors, such as applied bias and temperature.
- Conduction band: The conduction band is the energy band that electrons can occupy when they are free to move.
- Valence band: The valence band is the energy band that electrons can occupy when they are bound to atoms.
- Bandgap: The bandgap is the energy difference between the conduction band and the valence band.
- Fermi level: The Fermi level is the energy level at which the probability of finding an electron is 50%.
- Forward bias: When a forward bias is applied to a SiGe diode, the Fermi level moves closer to the conduction band, making it easier for electrons to flow from the n-type region to the p-type region.
- Reverse bias: When a reverse bias is applied to a SiGe diode, the Fermi level moves closer to the valence band, making it more difficult for electrons to flow from the n-type region to the p-type region.
- Breakdown voltage: The breakdown voltage is the voltage at which the reverse bias current suddenly increases. This is due to the fact that the electric field in the depletion region becomes so strong that it causes the diode to break down.
- Temperature dependence: The bandgap of a SiGe diode decreases with increasing temperature. This is because the thermal energy of the electrons increases with temperature, making it easier for them to overcome the bandgap.
The SiGe diode band diagram is a powerful tool for understanding the electrical properties of a SiGe diode. It can be used to design and optimize diodes for a variety of applications, such as solar cells, light-emitting diodes, and transistors.
Conduction band
In a SiGe diode, the conduction band is the energy band that electrons can occupy when they are free to move from the n-type region to the p-type region. The conduction band is separated from the valence band by the bandgap. When a forward bias is applied to the diode, the Fermi level moves closer to the conduction band, making it easier for electrons to flow from the n-type region to the p-type region.
- Electron transport: The conduction band plays a crucial role in the transport of electrons in a SiGe diode. When a forward bias is applied, electrons from the n-type region gain enough energy to overcome the bandgap and enter the conduction band. These electrons can then flow through the diode to the p-type region.
- Current-voltage characteristics: The conduction band is also responsible for the current-voltage characteristics of a SiGe diode. The forward bias current is determined by the number of electrons that can flow from the n-type region to the p-type region. The reverse bias current is determined by the number of electrons that can tunnel through the depletion region.
- Device performance: The conduction band can affect the performance of a SiGe diode. For example, the bandgap of a SiGe diode can be engineered to improve the efficiency of a solar cell.
The conduction band is an important part of the SiGe diode band diagram. It plays a crucial role in the transport of electrons, the current-voltage characteristics, and the device performance.
Valence band
In a SiGe diode, the valence band is the energy band that electrons can occupy when they are bound to atoms in the crystal lattice. The valence band is separated from the conduction band by the bandgap. When a reverse bias is applied to the diode, the Fermi level moves closer to the valence band, making it more difficult for electrons to flow from the n-type region to the p-type region.
The valence band plays a crucial role in the operation of a SiGe diode. Here are a few examples:
- Electron-hole recombination: When an electron in the conduction band recombines with a hole in the valence band, a photon is emitted. This process is essential for the operation of light-emitting diodes (LEDs).
- Tunneling: When a reverse bias is applied to a SiGe diode, electrons can tunnel through the depletion region from the valence band in the p-type region to the conduction band in the n-type region. This process is responsible for the reverse bias current in a SiGe diode.
- Device performance: The valence band can affect the performance of a SiGe diode. For example, the bandgap of a SiGe diode can be engineered to improve the efficiency of a solar cell.
The valence band is an important part of the SiGe diode band diagram. It plays a crucial role in the operation and performance of SiGe diodes.
Bandgap
In a SiGe diode, the bandgap is the energy difference between the conduction band and the valence band. The bandgap is an important property of a semiconductor material, as it determines the electrical and optical properties of the material.
- Electrical properties: The bandgap determines the electrical conductivity of a semiconductor material. A material with a large bandgap is a good insulator, while a material with a small bandgap is a good conductor.
- Optical properties: The bandgap also determines the optical properties of a semiconductor material. A material with a large bandgap is transparent to light, while a material with a small bandgap is opaque.
- Device performance: The bandgap of a SiGe diode can be engineered to improve the performance of the diode. For example, the bandgap of a SiGe diode can be engineered to improve the efficiency of a solar cell or the output power of a light-emitting diode.
The bandgap is a critical property of a SiGe diode. It determines the electrical and optical properties of the diode, and it can be engineered to improve the performance of the diode.
Fermi level
The Fermi level is a crucial concept in solid-state physics. It is the energy level at which the probability of finding an electron is 50%. In a semiconductor material, such as SiGe, the Fermi level lies within the bandgap, which is the energy difference between the conduction band and the valence band. The position of the Fermi level is determined by the doping concentration of the semiconductor material.
In a SiGe diode, the Fermi level plays an important role in determining the electrical properties of the diode. For example, the forward bias current is determined by the difference between the Fermi level in the n-type region and the Fermi level in the p-type region. The reverse bias current is determined by the difference between the Fermi level in the p-type region and the Fermi level in the n-type region.
The Fermi level is also important for understanding the operation of SiGe transistors. In a SiGe transistor, the Fermi level is used to control the flow of electrons from the source to the drain. By changing the gate voltage, the Fermi level can be moved closer to the conduction band or the valence band, which turns the transistor on or off.
The Fermi level is a critical concept for understanding the electrical properties of SiGe diodes and transistors. It is a key parameter that can be used to design and optimize these devices for a variety of applications.
Forward bias
This phenomenon can be clearly observed in the SiGe diode band diagram. When a forward bias is applied, the energy bands in the n-type and p-type regions bend, causing the Fermi level to move closer to the conduction band. This makes it easier for electrons to flow from the n-type region to the p-type region, as they have less energy to overcome.
- Energy band bending: When a forward bias is applied to a SiGe diode, the energy bands in the n-type and p-type regions bend. This bending is caused by the difference in electric potential between the two regions. The energy bands bend up in the n-type region and down in the p-type region.
- Fermi level movement: The Fermi level is the energy level at which the probability of finding an electron is 50%. When a forward bias is applied to a SiGe diode, the Fermi level moves closer to the conduction band. This movement is caused by the increase in the number of electrons in the conduction band.
- Electron flow: The movement of the Fermi level closer to the conduction band makes it easier for electrons to flow from the n-type region to the p-type region. This flow of electrons is what creates the forward bias current.
The forward bias characteristics of a SiGe diode can be tailored by changing the doping concentrations in the n-type and p-type regions. The doping concentration affects the position of the Fermi level, which in turn affects the energy band bending and the forward bias current.
Reverse bias
A reverse bias is a voltage applied to a diode that opposes the flow of current. In a SiGe diode, when a reverse bias is applied, the energy bands in the n-type and p-type regions bend in opposite directions. This bending causes the Fermi level to move closer to the valence band, making it more difficult for electrons to flow from the n-type region to the p-type region.
The si sige diode band diagram is a graphical representation of the energy bands in a SiGe diode. It can be used to visualize the effects of a reverse bias on the energy bands and the Fermi level. The band diagram shows that under reverse bias, the energy bands bend in opposite directions, and the Fermi level moves closer to the valence band. This makes it more difficult for electrons to flow from the n-type region to the p-type region, which is why a reverse bias is used to turn off a diode.
The reverse bias characteristics of a SiGe diode are important for understanding the operation of SiGe diodes in electronic circuits. By controlling the amount of reverse bias applied to a diode, the flow of current can be controlled. This is essential for the operation of many electronic devices, such as transistors, rectifiers, and voltage regulators.
Breakdown voltage
The breakdown voltage is an important parameter for SiGe diodes. It determines the maximum reverse bias voltage that can be applied to the diode before it breaks down. The breakdown voltage is also important for understanding the operation of SiGe diodes in electronic circuits. For example, in a voltage regulator circuit, the breakdown voltage of the diode determines the maximum output voltage of the circuit.
The breakdown voltage of a SiGe diode can be determined from the SiGe diode band diagram. The band diagram shows the energy bands in the SiGe diode under different bias conditions. Under reverse bias, the energy bands bend in opposite directions, and the electric field in the depletion region increases. As the reverse bias voltage is increased, the electric field in the depletion region becomes so strong that it causes the diode to break down.
The breakdown voltage of a SiGe diode can be controlled by changing the doping concentrations in the n-type and p-type regions. The doping concentration affects the width of the depletion region, which in turn affects the electric field in the depletion region. By controlling the doping concentrations, the breakdown voltage of the diode can be tailored to meet the requirements of a specific application.
The breakdown voltage of a SiGe diode is a critical parameter that must be considered when using SiGe diodes in electronic circuits. By understanding the relationship between the breakdown voltage and the SiGe diode band diagram, engineers can design and use SiGe diodes to optimize the performance of electronic circuits.
Temperature dependence
The bandgap of a semiconductor is the energy difference between the valence band and the conduction band. In a SiGe diode, the bandgap is typically around 1.1 eV at room temperature. However, the bandgap decreases with increasing temperature. This is because the thermal energy of the electrons increases with temperature, making it easier for them to overcome the bandgap.
The temperature dependence of the bandgap is an important factor to consider when designing SiGe diodes. For example, the forward bias voltage of a SiGe diode will decrease with increasing temperature. This is because the bandgap decreases with increasing temperature, making it easier for electrons to flow from the n-type region to the p-type region.
The temperature dependence of the bandgap can also be used to advantage in some applications. For example, SiGe diodes can be used as temperature sensors. By measuring the forward bias voltage of a SiGe diode, the temperature can be determined.
The temperature dependence of the bandgap is a fundamental property of semiconductors. It is important to understand this property when designing SiGe diodes and other semiconductor devices.
A SiGe diode band diagram is a graphical representation of the energy levels in a silicon-germanium (SiGe) diode. It shows the conduction band, valence band, and the bandgap between them. The band diagram can be used to understand the electrical properties of the diode, such as its forward bias and reverse bias characteristics.
SiGe diodes are important in many electronic applications, such as solar cells, light-emitting diodes (LEDs), and transistors. The band diagram can be used to design and optimize these devices for specific applications.
The main topics covered in this article include:
- The basics of SiGe diode band diagrams
- The importance of SiGe diode band diagrams
- The applications of SiGe diode band diagrams
FAQs on SiGe Diode Band Diagrams
SiGe diode band diagrams are an important tool for understanding the electrical properties of SiGe diodes. They can be used to design and optimize SiGe diodes for a variety of applications.
Question 1: What is a SiGe diode band diagram?
A SiGe diode band diagram is a graphical representation of the energy levels in a silicon-germanium (SiGe) diode. It shows the conduction band, valence band, and the bandgap between them.
Question 2: What are the applications of SiGe diode band diagrams?
SiGe diode band diagrams are used in the design and optimization of SiGe diodes for a variety of applications, including solar cells, light-emitting diodes (LEDs), and transistors.
Question 3: What are the advantages of using SiGe diode band diagrams?
SiGe diode band diagrams provide a clear and concise visualization of the energy levels in a SiGe diode. This makes them a valuable tool for understanding the electrical properties of SiGe diodes and for designing and optimizing SiGe diodes for specific applications.
Question 4: What are the limitations of SiGe diode band diagrams?
SiGe diode band diagrams are a simplified representation of the energy levels in a SiGe diode. They do not take into account all of the factors that can affect the electrical properties of a SiGe diode, such as temperature and doping concentration.
Question 5: How can I learn more about SiGe diode band diagrams?
There are a number of resources available to learn more about SiGe diode band diagrams. These resources include textbooks, journal articles, and online tutorials.
Question 6: How can I use SiGe diode band diagrams in my own work?
SiGe diode band diagrams can be used in a variety of ways, including:
- Designing and optimizing SiGe diodes for specific applications
- Understanding the electrical properties of SiGe diodes
- Teaching and learning about SiGe diodes
Summary of key takeaways or final thought
SiGe diode band diagrams are a valuable tool for understanding the electrical properties of SiGe diodes. They can be used to design and optimize SiGe diodes for a variety of applications.
Transition to the next article section
The next section of this article will discuss the applications of SiGe diode band diagrams in more detail.
Conclusion
The SiGe diode band diagram is a powerful tool for understanding the electrical properties of SiGe diodes. It can be used to design and optimize SiGe diodes for a variety of applications, including solar cells, light-emitting diodes (LEDs), and transistors.
In this article, we have explored the basics of SiGe diode band diagrams, their importance, and their applications. We have also discussed some of the limitations of SiGe diode band diagrams and how to learn more about them.
We encourage you to use SiGe diode band diagrams in your own work to design and optimize SiGe diodes for your specific applications.
Youtube Video:
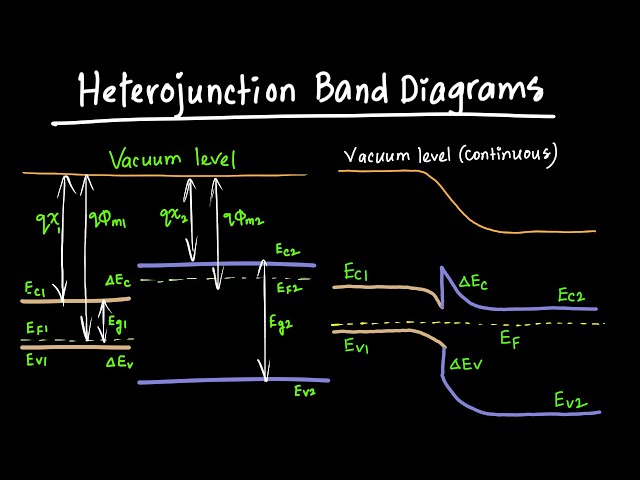